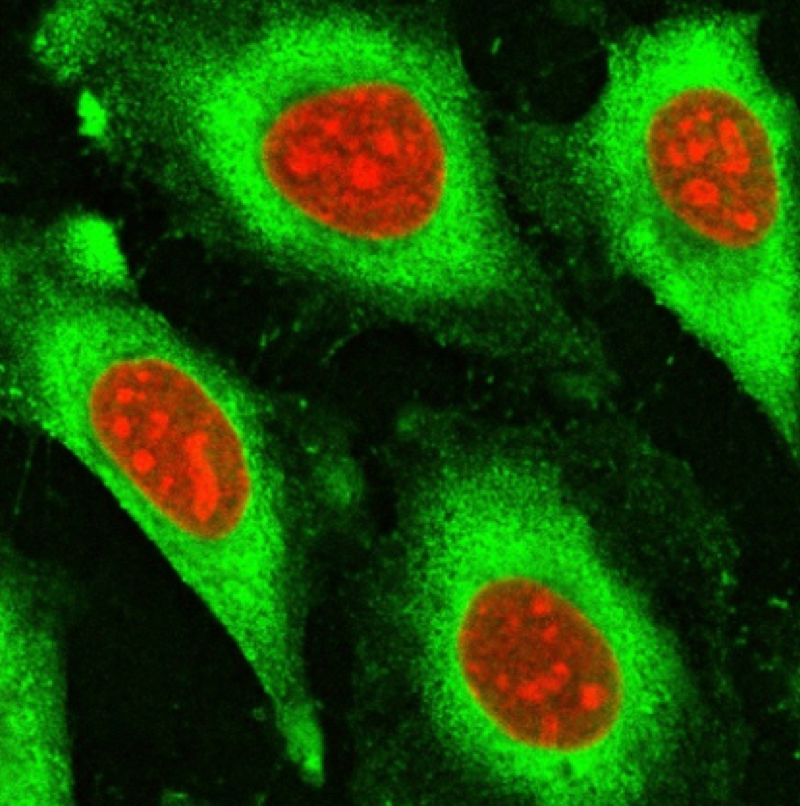
Cell-penetrating peptides (CPPs) are a class of short peptides that have the ability to facilitate the intracellular delivery of various molecular cargos. Among these, R9, a peptide consisting of nine arginine residues, has gained significant attention due to its efficiency in cellular uptake.
Key Takeaways:
- Cell-penetrating peptides (CPPs) are short peptides that can transport molecular cargos into cells.
- R9 is a type of CPP that consists of nine arginine residues and is known for its efficient cellular uptake.
The Science Behind R9
What is R9?
The R9 peptide is a type of cell-penetrating peptide that is characterized by its nine arginine residues. Arginine is a cationic amino acid, which means it carries a positive charge. This property allows R9 to interact with the negatively charged cell membrane, facilitating its penetration into the cell.
How Does R9 Work?
Like other CPPs, R9 can translocate across the plasma membrane and facilitate the intracellular delivery of various cargos. These cargos can range from small molecules and peptides to larger entities such as proteins and nucleic acids. R9’s ability to deliver these cargos into cells has opened up new possibilities in the fields of drug delivery and cellular biology.
Applications of R9
Drug Delivery
One of the most promising applications of R9 is in the field of drug delivery. By attaching therapeutic molecules to R9, researchers can enhance the cellular uptake of these therapies, improving their efficacy. For example, R9 has been used to deliver anti-cancer drugs into tumor cells, enhancing the effectiveness of cancer treatment.
Discover the potential of TAT peptides in drug delivery at LifeTein’s peptide synthesis services.
Genetic Engineering
R9 also has potential applications in genetic engineering. In one study, researchers used R9 to deliver DNA into plant tissues, demonstrating the potential of R9-mediated transfection in plant biotechnology (read the study)

Challenges in Using R9
Stability Issues
One of the challenges in using R9 and other CPPs is their stability. These peptides are susceptible to degradation by proteases, enzymes that break down proteins and peptides. This can limit the effectiveness of R9-mediated delivery of cargo.
Off-Target Effects
Another challenge is the potential for off-target effects. Because R9 and other CPPs can enter all types of cells, there is a risk that they could deliver their cargo to the wrong cells, leading to unintended effects.
Future Prospects
Despite these challenges, the future of R9 and other CPPs in biomedical research and applications looks promising. Researchers are developing strategies to improve the stability of these peptides and to target them more specifically to the desired cells. With these advancements, R9 and other CPPs could revolutionize drug delivery and genetic engineering fields.
For more information on peptide-based therapies and CPPs, explore LifeTein’s services.
Frequently Asked Questions
- What are Cell Penetrating Peptides (CPPs)?
- Cell Penetrating Peptides (CPPs) are short peptides that can transport molecular cargo into cells.
- What is R9?
- R9 is a type of CPP consisting of nine arginine residues, and it is known for its efficient cellular uptake.
- How does R9 work?
- R9, like other CPPs, can translocate across the plasma membrane and facilitate the intracellular delivery of various cargos.
- What are the applications of R9?
- R9 has promising applications in the fields of drug delivery and genetic engineering.
Wu, H., Zhang, Z., Zhu, K., Wang, Y., Ke, F., Zhang, F., Zhao, Y., Zhang, Y., & Feng, H. (2022). Cell-penetrating peptide-mediated transfection of proteins into tissues of Arabidopsis and Chinese cabbage. In In Vitro Cellular & Developmental Biology – Plant (Vol. 58, Issue 1, pp. 28–34). Springer Science and Business Media LLC. https://doi.org/10.1007/s11627-021-10221-0