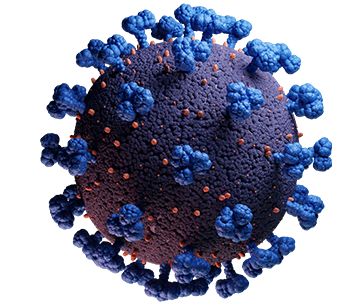
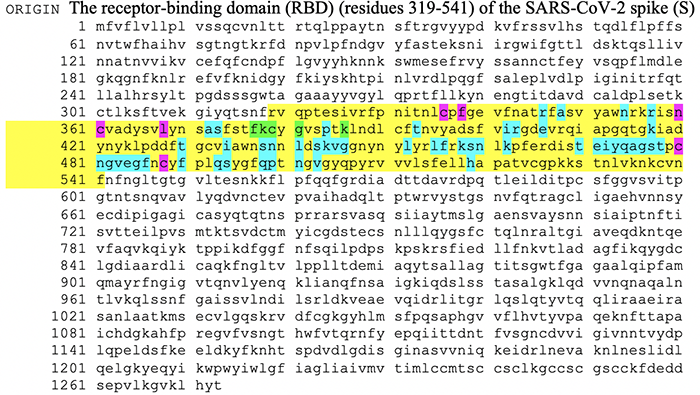
LifeTein can help in your research with custom peptide synthesis of the following specific proteins: SARS-CoV-2 Receptor Binding Domains, SARS-CoV-2 Nucleocapsid Fragments, T-cell and B-cell Epitopes of SARS-CoV-2, Fusion Inhibitors Targeting HR1 Domain of the SARS-CoV-2 Spike Proteins, Inhibitors of SARS-CoV-2 Mpro/3CLpro/C30 Endopeptidase, ACE2 Inhibitors and Substrates, and AT2 Receptor Agonists and Antagonists.
Pool of 22 peptides derived from a peptide design (15mers with 5 aa overlap) through the receptor binding domain of S1 protein.
Modifications: N-Terminal: Biotin Labeling
Amount: 1mg per peptide
Purity: 95%
Delivery Format: Freeze dried powder
Application(s): Antibody screening, T-cell assays, Immune monitoring, Antigen specific T-cell stimulation, Cellular immune response
Indication(s)/Topic(s): Covid-19, Infection, Respiratory infection
Delivery Time: 2 weeks
SARS-CoV-2 Receptor Binding Domains Overlapping Peptide Pools:
- QPTESIVRFPNITNL
- NITNLCPFGEVFNAT
- VFNATRFASVYAWNR
- YAWNRKRISNCVADY
- CVADYSVLYNSASFS
- SASFSTFKCYGVSPT
- GVSPTKLNDLCFTNV
- CFTNVYADSFVIRGD
- VIRGDEVRQIAPGQT
- APGQTGKIADYNYKL
- YNYKLPDDFTGCVIA
- GCVIAWNSNNLDSKV
- LDSKVGGNYNYLYRL
- YLYRLFRKSNLKPFE
- LKPFERDISTEIYQA
- EIYQAGSTPCNGVEG
- NGVEGFNCYFPLQSY
- PLQSYGFQPTNGVGY
- NGVGYQPYRVVVLSF
- VVLSFELLHAPATVC
- PATVCGPKKSTNLVK
- TNLVKNKCVNFNFNG