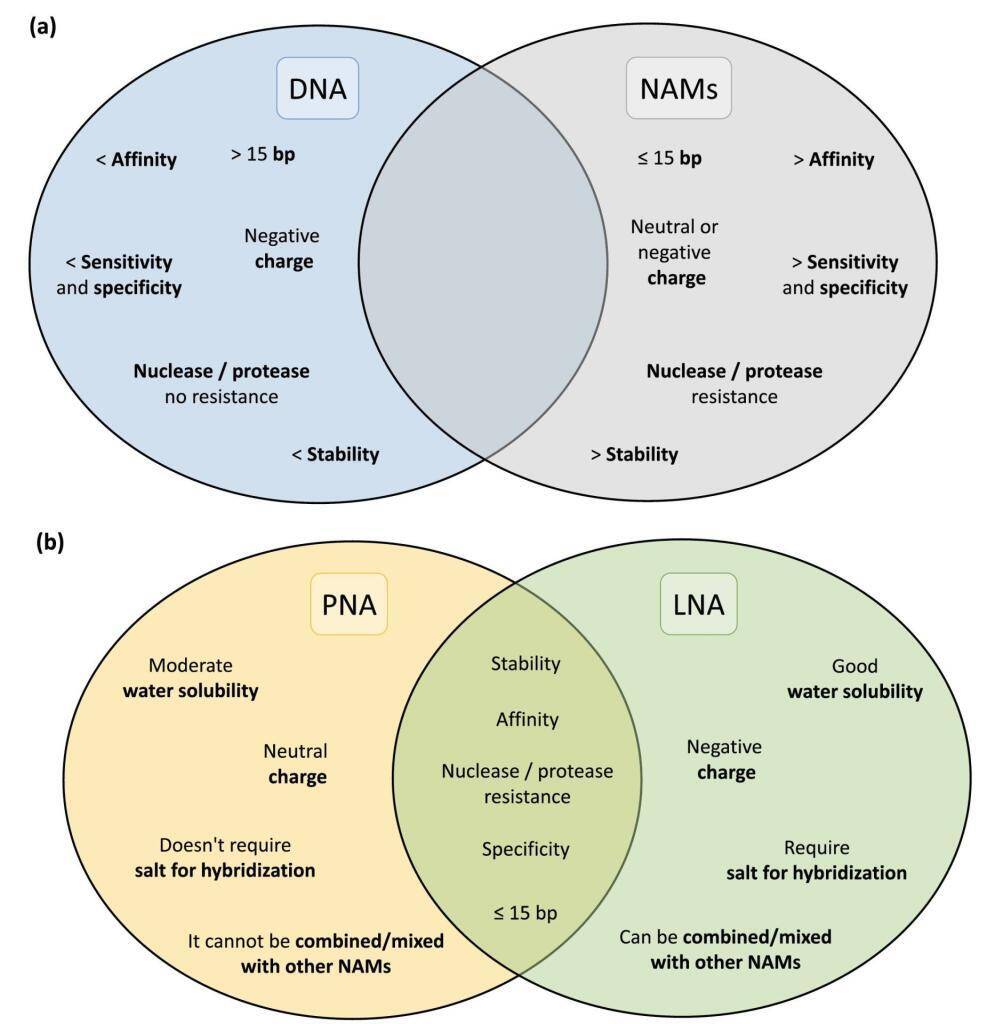
The landscape of in situ hybridization (ISH), specifically fluorescence in situ hybridization (FISH), has undergone a transformative shift with the introduction of Nucleic Acid Mimics (NAMs). These modified probes, encompassing Peptide Nucleic Acid (PNA), Locked Nucleic Acid (LNA), 2′-O-Methyl-RNA, UNA (unlocked nucleic acid), and Phosphorodiamidate Morpholino Oligomers (PMOs), have emerged as groundbreaking tools, overcoming the limitations associated with traditional DNA and RNA probes.
Peptide Nucleic Acids (PNAs):
Peptide Nucleic Acids (PNAs) stand at the forefront of this molecular revolution, offering a fusion of DNA specificity and peptide versatility. The distinctive PNA backbone, composed of peptide linkages, ensures unparalleled stability and resistance to enzymatic degradation. With superior hybridization properties, PNAs bind to complementary DNA or RNA sequences with exceptional affinity, making them indispensable for applications ranging from targeted gene therapy to diagnostic assays and antisense technologies.
Unlocking the Potential: PNA’s Key Features:
Stability and Resistance:
PNAs, characterized by a neutral polyamide backbone, showcase remarkable stability against nucleases and enzymatic degradation. This attribute enhances the half-life of PNA molecules, ensuring their efficacy in diverse experimental conditions.
High-Affinity Binding:
The hybridization capabilities of PNAs are unparalleled, facilitating strong, sequence-specific binding to target nucleic acids. PNA’s shorter length allows for enhanced cell penetration and consistent hybridization performance, even under low salt concentrations. Its unique melting temperature response to single nucleotide changes enables precise probe design.
Versatility in Applications:
PNAs find applications across a spectrum of research areas, including molecular diagnostics, gene editing, and nanotechnology. Their adaptability for specific sequences and functions makes PNAs an invaluable asset in the molecular biologist’s toolkit.
Locked Nucleic Acid (LNA):
Described in 1997, Locked Nucleic Acid (LNA) boasts a ribose ring locked in a specific conformation, ensuring water solubility and low toxicity. LNA’s design flexibility, including modifications like phosphorothioate, enhances resistance to nucleases without compromising affinity. Combining LNA with 2′-O-Methyl-RNA provides flexibility in melting temperature adjustments for optimized hybridization efficiency.
UNA and Other NAMs:
UNA, an acyclic RNA analog, offers flexibility, though it may impact nucleic acid duplex stability. Modifications like 2′-pyrene and 3′-O-amino-UNA address stability concerns, presenting potential applications in FISH experiments. Additionally, Phosphorodiamidate Morpholino Oligomers (PMOs), characterized by non-ionic properties and resistance to nucleases, have shown success in bacterial and fungal infection detection via FISH.
Challenges and Progress:
Despite the exceptional qualities of NAMs, particularly PNA and LNA, their widespread adoption in FISH for microorganism detection has been slower than anticipated. Challenges include hydrophobicity and water solubility issues for PNA probes, along with a general lack of awareness among laboratories. Nevertheless, studies showcasing PNA’s superior performance over traditional DNA probes underscore the promising potential of NAMs in microbial detection.
Conclusion:
As research in the field progresses, ongoing efforts are addressing the limitations associated with NAMs. Their unique features position them as compelling alternatives for FISH-based microorganism detection, holding the promise of unlocking new frontiers in advanced genetic research.